A major challenge facing 5G deployment is that the available sub-6 GHz spectrum does not support the latency and throughput needed to deliver the optimal performance required by advanced applications and simultaneous users. While current sub-6 GHz 5G networks provide marginal improvements over existing 4G LTE networks, they fail to deliver on the promise of 5G coverage, performance and latency in dense urban environments and crowded event venues.
5G networks using mmWave frequencies in the 24 to 40 GHz range hold the most promise for high bandwidth, low latency 5G connectivity. 5G mmWave networks provide orders of magnitude improvement in throughput and capacity because of the availability of wider spectrum bands at 28, 39 and 60 GHz frequencies and massive frequency reuse with highly directional antennas.
The emergence of next-generation 5G networks in the mmWave bands, however, has introduced technical challenges as well. Deployment of mmWave technology poses an array of signal propagation, blocking and path loss challenges that need to be overcome:
1. Higher propagation loss at high carrier frequencies — As carrier frequencies increase, signal power decreases with a single antenna of size ∼λ/2, i.e., where the length of an antenna is approximately equal to half the wavelength, λ. At mmWave frequencies, an antenna is smaller than at low- or mid-band frequencies, so its area scales with the square of the wavelength. The power received scales accordingly. An antenna array compensates for this scaling, but the signal phases must be aligned through a technique called beamforming.
2. The need for a trackable LOS path or a strong reflective path between the transmitter and receiver — mmWave signals are typically either LOS or reflected from objects. Mainly because the wavelength is small compared to the edges or roughness of objects, there is less scattering. The lack of refraction and diffraction in high frequency radio signals—due to targeted beamforming—limits the availability of multiple links to the LOS path or strong mirror-like reflective paths. This is a major constraint in delivering stable wireless connections that must be available anywhere, anytime.
Beamforming combined with beam tracking and beam management algorithms provide a robust link. With 5G mmWave networks, since both polarizations are initiated from the same antenna, and since objects and walls mainly reflect the beam, the same beam carries both polarizations independently to support 2 x 2 MIMO. This is not the case at lower frequencies.
3. High transmittance loss through materials at high radio frequencies — Compared to sub-6 GHz, mmWave signals demonstrate very high losses when propagating through typical materials, such as glass, tinted glass, wood and drywall. This is an additional constraint on signal availability and stability.
For mmWave signal, the high propagation loss is well understood. For the same capture area, the path loss can be compensated with a commonly accepted solution. It is addressed by using steerable phased arrays and by building large antenna apertures through the co-phasing of many small antenna elements. However, there are no widely agreed upon or standard mitigation techniques to address the second and third challenges, although we have seen similar technical challenges with satellite TV and Wi-Fi. These have been addressed with additional access points, boosters, repeaters and satellite alignment.
DEPLOYMENT CHALLENGES
Consider three deployment scenarios in which a repeater solution can be designed to improve link availability:
Lack of LOS or a Strong Reflective Path
Figure 1 illustrates a case where there is no LOS or strong reflective path between the gNB base station and the user equipment (UE). Given the reflective propagation properties at high frequencies, natural or passive reflectors must create a mirror-like path between the source and destination. Without a repeater, as shown, this limits deployment to LOS scenarios or those that can rely on natural mirrors in the environment to close the link.
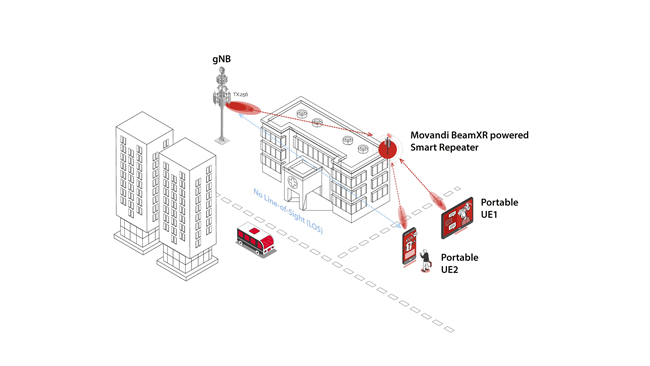
Figure 1 Propagation environment lacking LOS.
Very High Transmittance Loss
Figure 2 illustrates a deployment scenario with a very high transmittance loss due to objects between the gNB and UE. For example, transmission loss through tinted glass can be as high as ∼40 dB, which is challenging to budget for in a single link hop.
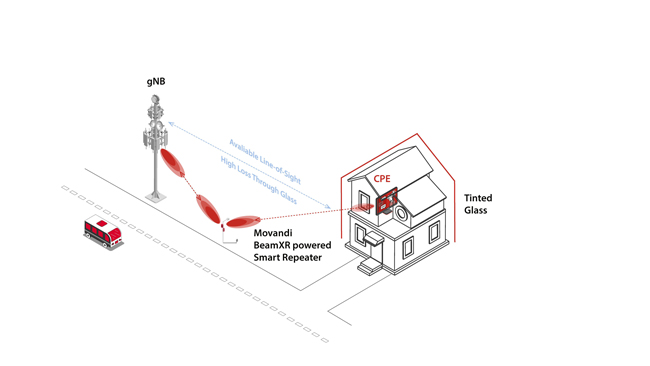
Figure 2 Propagation environment suffering from very high transmittance loss.
Untrackable Changing Environment and/or Reflectors
Ideally, beam tracking algorithms track and adapt to movements and changes in the environment, reflectors and UE. However, typical changes in the environment, such as blockage of LOS or a change in orientation of the UE, may prove difficult to track without losing the connection. So more mitigation methods and architectural improvements are required to provide robust, reliable connections for mobile and varying environments (see Figure 3).
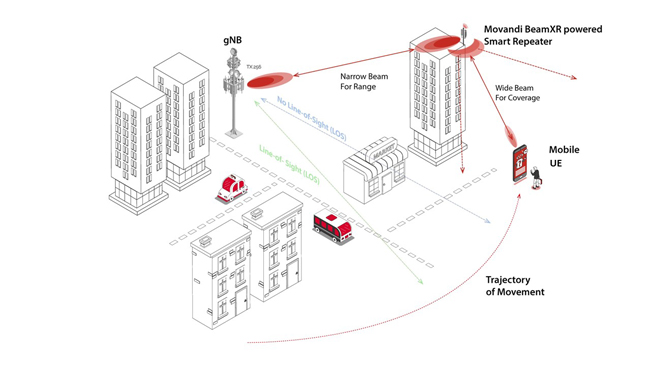
Figure 3 Mobile propagation environment with challenging untrackable paths and reflectors.
SMART REPEATER ARCHITECTURE
Active repeaters can be designed to improve mmWave link availability and address LOS challenges. To minimize latency, cost and complexity, a repeater based on a “demodulator-less” architecture maximizes the available signal strength and eliminates the need for conventional demodulation and remodulation techniques.
Figure 4 shows three different variations of this architecture. The RF-only repeater has negligible latency and the lowest loss, yet it may amplify adjacent channels. The RF-analog repeater has negligible latency and can suppress the adjacent channels before transmission. The smart repeater suppresses the adjacent channels and provides the opportunity for sample-level manipulation of the signal without incurring noticeable latency. It may use, for example, digital predistortion for the power amplifier and digital post or pre-equalization to compensate for non-flat responses of the RF and analog blocks.
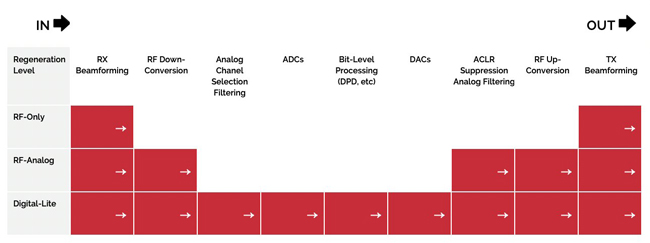
Figure 4 Demodulator-less repeater variations.
A smart repeater deployed between the gNB and UE can mitigate the lack of LOS between the gNB and UE. Figure 5 summarizes the results for the deployment scenario shown in Figure 1, with and without a repeater.
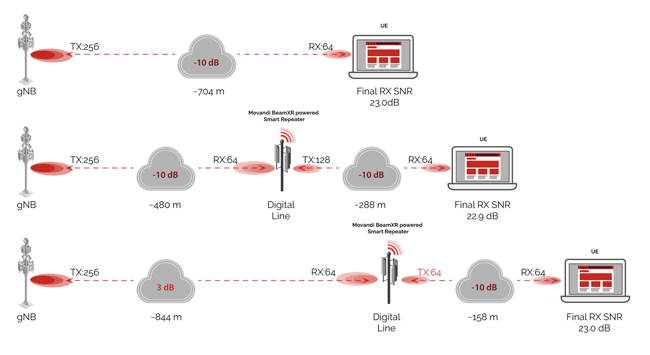
Figure 5 Using a repeater to address the lack of LOS.
The original article website:
https://www.microwavejournal.com/articles/38666-optimizing-active-repeater-architectures-for-distributed-5g-networks